Genomics: Insight
The underlying genetic causes of pulmonary fibrosis: a systematic review
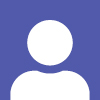
Research Question: What role do genetic factors play in the development and treatment of pulmonary fibrosis?
Introduction
What role do genetic factors play in the development and treatment of pulmonary fibrosis? Pulmonary fibrosis (PF) is the most common form of interstitial lung disease (ILD), impacting approximately 35,000 to 55,000 Americans each year4. PF is characterized by the progressive scarring and thickening of the interstitial tissue between the alveoli, the tiny air sacs responsible for gas exchange. This scarring interferes with the lungs’ ability to take in oxygen and expel carbon dioxide waste, leading to shortness of breath and chronic coughing. Understanding the genetic influences of PF is key to uncovering its underlying causes.
PF typically affects middle-aged to older men and leads to an average life expectancy of up to five years following diagnosis18. Diagnosis is often confirmed through tests like the predicted Forced Vital Capacity (FVC) and predicted Diffusing Capacity of the Lung for Carbon Monoxide (DLCO), which assess overall lung function24.
While genetic mutations are significant contributors to PF susceptibility, they do not act in isolation. Environmental exposures, such as smoking, occupational hazards, and particulate matter, can exacerbate disease progression, especially in individuals with underlying genetic vulnerabilities22. These environmental factors may interact with genetic mutations to accelerate epithelial damage, compounding the disease process22. This interplay between genetic and environmental factors is central to understanding PF’s multifaceted etiology and emphasizes the need for integrated research to advance targeted treatments.
PF can develop either sporadically or hereditarily, with a 30% increased risk if two or more family members within three degrees of relationship have PF7. Initially, PF may resemble other forms of ILD, characterized by milder symptoms, which can delay diagnosis until lung function has significantly deteriorated19. About half of PF cases are classified as idiopathic pulmonary fibrosis (IPF), where the cause of the disease remains largely unknown.
Genetic causes of pulmonary fibrosis
The possibility of a gene controlling PF and its transmission across generations was first suggested in the 1950s when it was detected in identical twins16. It is now known that familial PF exhibits autosomal dominant inheritance and involves multiple genetic components working together.
To date, there are over 20 known mutations that can be categorized under two main genetic causes of PF—single nucleotide polymorphisms and shortened telomeres.
Single nucleotide polymorphisms (SNPs) of PF
Single nucleotide polymorphisms (SNPs) are genetic mutations in which one base in a gene sequence is substituted with another base. Many SNPs that target surfactant proteins have been linked to PF. Surfactant proteins are responsible for maintaining lung surface homeostasis by clearing various bacteria and fungi and reducing inflammation in the tissue7. These mutations cause an increase in tissue scarring and fibroblast formation. One of the common examples of an SNP causing PF is that of MUC5B. The MUC5B gene encodes for the MUC5B protein, which is responsible for mucus formation and maintenance of a healthy respiratory tract. This SNP is responsible for 30-40% of PF cases and is one of many genes factored into the familial and sporadic model of PF5.
Telomeres and telomerases
Telomeres are stabilizing regions at the end of DNA that prevent the chromosomes from degradation or losing genes while dividing. During DNA replication and cell division, telomeres continuously shorten until they are too short for the cell to continue dividing. Then, the divided cell undergoes apoptosis and dies7. Cells which regularly divide, such as epidermal cells, have an enzyme called telomerase, which helps maintain telomere length and prolongs cell life. However, when the gene that codes for telomerase is mutated, cells often die rapidly and prematurely, greatly impacting various tissues21.
There are several telomere mutations associated with PF, including mutations in the telomerase RNA component, (TERC), and mutations in the telomerase reverse transcriptase gene (the TERT gene)3. TERC and TERT work together to maintain telomere length, where TERC acts as a template for telomere DNA, and TERT adds new DNA sequences to the ends of chromosomes13. Mutated telomere length is associated with a shorter lifespan and 20-30% of familial pulmonary fibrosis cases9.
Telomere shortening, also known as telomeropathy, along with genetic mutations, causes DNA damage over time. This leads to apoptosis (cell death) and poor tissue repair in the pulmonary epithelium, causing permanent scarring and the development of pulmonary fibrosis. Loss of telomerase function has further affects in other high-turnover tissues in the body, such as the GI tract and bone marrow23.
Considering gene therapies to treat pulmonary fibrosis
Although many gene therapy options have been proposed for treating pulmonary fibrosis, none have yet been tested in clinical trials. A variety of factors have contributed to this, including the lack of animal models that accurately replicate human PF.
Gene-based therapies introduce exogenous, or external, nucleic acids into cells to undo the effects of both loss and gain-of-function mutations1. Gene therapy can be executed using either viral or non-viral vectors. Generally, viral vectors utilize modified viruses to introduce and replicate new nucleic acids in cells. Non-viral vectors use physical and chemical techniques such as polymers and injection to directly deliver genetic material into the cell. With regards to PF, both vectors would look to target the TERT gene, either through replacing it or editing it using gene technology such as CRISPR-Cas912. Restoring the TERT gene’s function could potentially restore the lung cells’ ability to repair and regenerate, mitigating the disease progression.
Non-viral vectors are generally favored over viral ones because they cause a smaller immune response and are easier to produce. However, the mucus in the airways contains negatively charged particles that can bind to these vectors, leading to their destabilization and destruction1. Additionally, macrophages in the alveoli and intracellular structures like lysosomes can further inhibit their effectiveness5. Although there are various types of non-viral vectors, such as lipids, polymers, and proteins, that could be used for this purpose, no clinical trials or commercial products utilizing these therapies are currently available.
When weighing potential therapies for PF, the administration of drugs through the nose or mouth is preferred to system-wide methods1. Many pathogenic molecules present in PF are also responsible for other crucial bodily functions, and a generalized delivery can have off-target effects.
Although many gene therapy options have been proposed for treating pulmonary fibrosis, none have yet been tested in clinical trials.
Summary
PF is a complex disease, strongly influenced by genetic factors. Specific genetic variations, such as SNPs in surfactant proteins and the MUC5B gene, along with mutations in the TERT and TERC genes that impact telomere maintenance, play a crucial role in the development of this disease. These mutations contribute to lung tissue scarring and the overgrowth of fibroblasts, ultimately leading to reduced lung function.
Gene therapies targeting these genetic factors, particularly using CRISPR-Cas9 to address TERT mutations, present a promising treatment approach. However, physiological barriers such as degradation by mucus and uptake by macrophages in the lung tissue need to be overcome before effective delivery mechanisms in clinical applications can be considered. Non-viral vectors are preferred because they elicit less immune response and are a more targeted approach for treating the lung as opposed to systemic administration1.
Despite these challenges, ongoing research holds promise for developing more effective PF treatments and moves us closer to personalized medicine for PF patients.
References:
- Huang, Teng, Jia Gao, Long Cai, Hao Xie, Yuhan Wang, Yi Wang, and Qing Zhou. 2022. “Treating Pulmonary Fibrosis with Non-Viral Gene Therapy: From Bench to Bedside.” Pharmaceutics, 14 (4).
- Rojas, M., & Mora, A. L. (2022). “Idiopathic Pulmonary Fibrosis: Update on Genetics and Telomeres.” American Journal of Respiratory and Critical Care Medicine.
- Newton, Chad A., Kiran Batra, Jose Torrealba, Julia Kozlitina, Craig S. Glazer, Carlos Aravena, Keith Meyer, Ganesh Raghu, Harold R. Collard, and Christine Kim Garcia. 2016. “Telomere-Related Lung Fibrosis Is Diagnostically Heterogeneous but Uniformly Progressive.” The European Respiratory Journal: Official Journal of the European Society for Clinical Respiratory Physiology, 48 (6): 1710–20.
- Borie, Raphael, Laure Tabèze, Gabriel Thabut, Hilario Nunes, Vincent Cottin, Sylvain Marchand-Adam, Grégoire Prevot, et al. 2016. “Prevalence and Characteristics of TERT and TERC Mutations in Suspected Genetic Pulmonary Fibrosis.” The European Respiratory Journal: Official Journal of the European Society for Clinical Respiratory Physiology, 48 (6): 1721–31.
- Ryu, J. H., & Moua, T. (2020). “The Impact of Telomere Biology on Idiopathic Pulmonary Fibrosis.” American Journal of Respiratory and Critical Care Medicine, 201(10), 1187–1197.
- Dratwa, Marta, Barbara Wysoczańska, Piotr Łacina, Tomasz Kubik, and Katarzyna Bogunia-Kubik. 2020. “TERT-Regulation and Roles in Cancer Formation.” Frontiers in Immunology, 11 (November): 589929.
- Gooptu, Bibek. 2022. “Surfactant Protein C Mutations and Familial Pulmonary Fibrosis: Stuck in a Loop on the Scenic Route.” The European Respiratory Journal: Official Journal of the European Society for Clinical Respiratory Physiology, 59 (1): 2102147.
- Chadwick, Lisa H. n.d. “Telomere.” Genome.gov. Accessed August 22, 2024.
- Cong, Yu-Sheng, Woodring E. Wright, and Jerry W. Shay. 2002. “Human Telomerase and Its Regulation.” Microbiology and Molecular Biology Reviews: MMBR, 66 (3): 407–25.
- Pulmonary Fibrosis Foundation. (n.d.). “Genetic Testing in Pulmonary Fibrosis for Health Care Providers.” Position Statements.
- Somogyi, V., Chaudhuri, N., Torrisi, S. E., Kahn, N., Müller, V., & Kreuter, M. (2019). The therapy of idiopathic pulmonary fibrosis: what is next? European Respiratory Review, 28(153), 190021. https://doi.org/10.1183/16000617.0021-2019
- Borie, Raphael, Laure Tabèze, Gabriel Thabut, Hilario Nunes, Vincent Cottin, Sylvain Marchand-Adam, Grégoire Prevot, et al. 2016. “Telomere Length and Pulmonary Fibrosis.” The European Respiratory Journal: Official Journal of the European Society for Clinical Respiratory Physiology, 48 (6): 1721–31.
- Papiris, Spyros A., Caroline Kannengiesser, Raphael Borie, Lykourgos Kolilekas, Maria Kallieri, Vasiliki Apollonatou, Ibrahima Ba, et al. 2022. “Genetics in Idiopathic Pulmonary Fibrosis: A Clinical Perspective.” Diagnostics (Basel, Switzerland), 12 (12): 2928.
- Planas-Cerezales, Lurdes, Elena G. Arias-Salgado, Cristina Berastegui, Ana Montes-Worboys, Rafaela González-Montelongo, José M. Lorenzo-Salazar, Vanesa Vicens-Zygmunt, et al. 2021. “Lung Transplant Improves Survival and Quality of Life Regardless of Telomere Dysfunction.” Respiratory Research, 22 (1): 98.
- Klay, D., Grutters, J. C., van der Vis, J. J., Platenburg, M. G. J. P., Kelder, J. C., Tromp, E., & van Moorsel, C. H. M. (2023). Progressive disease with low survival in adult patients with pulmonary fibrosis carrying surfactant-related gene mutations: An observational study. Chest, 163(4), 870–880.
- Zisman, D. A., Keane, M. P., Belperio, J. A., Strieter, R. M., & Lynch, J. P., 3rd. (2005). Pulmonary fibrosis. Methods in Molecular Medicine, 117, 3–44.
- SFTPC surfactant protein C [Homo sapiens (human)] - Gene - NCBI. (n.d.). Accessed August 22, 2024, from https://www.ncbi.nlm.nih.gov/gene/6440
- Idiopathic pulmonary fibrosis (IPF). (2024, August 2). Accessed August 22, 2024, from https://emedicine.medscape.com/article/301226-overview
- What are interstitial lung diseases? (n.d.). NHLBI, NIH. Accessed August 22, 2024, from https://www.nhlbi.nih.gov/health/interstitial-lung-diseases
- GAP index for idiopathic pulmonary fibrosis (IPF) mortality. (n.d.). MDCalc. Accessed August 22, 2024, from https://www.mdcalc.com/calc/2157/gap-index-idiopathic-pulmonary-fibrosis-ipf-mortality
- TERT gene. (n.d.). Accessed August 22, 2024, from https://medlineplus.gov/genetics/gene/tert/
- Gandhi, S., Tonelli, R., Murray, M., Samarelli, A. V., & Spagnolo, P. (2023). Environmental causes of idiopathic pulmonary fibrosis. International Journal of Molecular Sciences, 24(22), 16481. https://doi.org/10.3390/ijms242216481
- Shay, J. W., & Wright, W. E. (2012). Telomeres and age-related diseases: How telomere biology informs clinical paradigms. The Journal of Clinical Investigation, 122(10), 3159–3168. https://doi.org/10.1172/JCI66370
- Jones, R., & Richeldi, L. (2022). Idiopathic pulmonary fibrosis: A review of the clinical and epidemiological aspects. The Lancet Digital Health, 4(9), E605–E617. https://doi.org/10.1016/S2589-7500(22)00173-X
About the Author
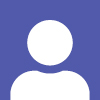
Saachi Jain is a senior at Saratoga High School. As a research intern at Stanford's Snyder Lab, she worked on metabolomics and exposomics. Her interest in pulmonary fibrosis arose when her grandfather was diagnosed with the condition in 2018. She enjoys cooking, music, and playing with her cats.
Mentor: Dr.Xiangping Lin. Affiliation: Stanford University