Genomics: Insight
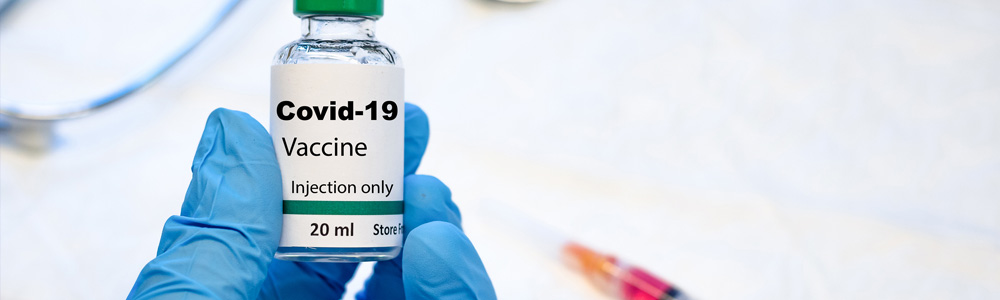
A Look into the Creation of a COVID-19 Vaccine
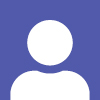
In December 2019, COVID-19, a new respiratory disease, broke out in Wuhan, China, resulting in a worldwide pandemic. A vaccine is needed, but what does vaccine creation entail?
What is COVID-19?
COVID-19 is a zoonotic disease caused by the virus SARS-CoV-2, which is a type of coronavirus with a genome size of 30 kilobases. The structural proteins of the virus include the spike protein, membrane protein, envelope protein, and nucleocapsid protein. Viral replication utilizes all structural proteins and infection of the host involves binding the spike protein to the angiotensin-converting enzyme II (ACEII) (Ahmed).
How do vaccines work?
Vaccines use the target antigen, the molecular structure that elicits an antibody response, to create antigen-specific immunity. Vaccines can be live-attenuated (a weakened form of the antigen), inactivated (an inactive form of the antigen), or a subunit (a part of the antigen). The use of a live-attenuated vaccine easily provides strong immunity but cannot be administered to weak immune systems, as the antigen is still harmful. Alternatively, an inactivated vaccine can be administered to weak immune systems because it cannot cause illness, but it is less potent and usually requires many doses to achieve immunity. Using only a part of the antigen, like a viral protein, creates a vaccine that can induce immunity after two doses while still being safe enough for weaker immune systems. But subunit vaccines often require an adjuvant to boost the immune response to the antigen. Additionally, thanks to modern gene-editing techniques, scientists can create vaccines that use genetic material. The recombinant vaccine is one such example. It inserts the antigen’s genetic material into a vector so that the genes can be expressed from the vector and purified. The resulting protein is used in the vaccine. DNA and RNA vaccines also utilize genetic material. These vaccines directly inject the antigen’s DNA or RNA into the recipient, so that the recipient’s cells can express the genes (“Vaccine Types”). However, the genetic material needs to be encapsulated in a vector, because raw DNA and RNA degrade in biological fluids (Cullis and Hope).
“Vaccines use the target antigen to create antigen-specific immunity.”
Where do we start?
Unfortunately, not much is known about SARS-CoV-2, so vaccine creation is difficult. But the virus that causes SARS, SARS-CoV, is genetically similar to SARS-CoV-2. As a result, immunological information concerning SARS can be used in the COVID-19 vaccine investigation (Ahmed).
For example, one can note the significance of the spike and nucleocapsid proteins. The spike proteins make up the outermost membrane of the virus, and the nucleocapsid proteins make up the capsid, or the casing, around the viral genetic material. Targeting spike proteins in SARS vaccines has provoked strong immune responses, likely because of the role that spike proteins play in binding the virus to the host. Targeting nucleocapsid proteins also provoked strong immune responses, which bodes well because the protein is abundant during infection. These two proteins serve as a good starting point for COVID-19 vaccine research (Ahmed).
The roles of humoral (B-cell) and cell-mediated (T-cell) immune responses in the case of SARS are also insightful. In SARS, cell-mediated immune responses appears to be the most powerful, especially those that target spike and nucleocapsid proteins. Several of these T-cell epitopes from SARS-CoV are also present on SARS-CoV-2. Humoral immune responses could potentially be of interest as well: SARS-CoV B-cell responses against spike proteins in mice were able to protect against SARS, and many B-cell epitopes from SARS-CoV map onto SARS-CoV-2 perfectly. There are successful SARS B-cell responses that target the nucleocapsid protein, but the immunity does not last long (Ahmed).
What are some of the vaccine options right now, and how do they work?
Clinical trials have begun for five potential vaccines as of April 8, all of which somehow utilize genomics (Le).
One vaccine was manufactured by ModernaTX Inc. and is called mRNA-1273. It is an mRNA vaccine that encodes for a SARS-CoV-2 spike protein (“Safety and Immunogenicity Study of 2019-nCoV Vaccine”. The mRNA is encapsulated for injection in a lipid nanoparticle (LNP), which is a non-viral vector that is 100 nanometers or less in size and can maintain stability for at least a year at 4 degrees C (Cullis and Michael).
“Clinical trials have begun for five potential vaccines as of April 8, all of which utilize genomics.”
Cansino Biologics Inc. is also investigating their own COVID-19 vaccine, called Ad5-nCoV. Theirs is a recombinant vaccine that expresses the spike protein, like the vaccine above. This vaccine utilizes an adenovirus type 5 vector for the genetic material (“Phase 1 Clinical Trial of a COVID-19 Vaccine”). An adenovirus is a virus that is a potent viral vector because of its high immunogenic properties and large and easily edited genome (about 36 kilobases) (Zhang and Zhou).
Another potential vaccine is INO-4800, from Inovio Pharmaceuticals. It is a DNA plasmid vaccine that also encodes for the spike protein (“Safety, Tolerability, and Immunogenicity of INO-4800”). A DNA plasmid is a non-chromosomal circular DNA molecule that is used in DNA vaccines as a vector for the genetic material (“DNA Vaccines”). This vaccine uses electroporation, which is a method of genetic vaccine delivery that involves the use of a pulse of electricity to open the pores in the cell membrane, making the administration of the vaccine more efficient (Sardesai and Weiner).
LV-SMENP-DC is another vaccine from the developer Shenzhen Geno-Immune Medical Institute. It uses dendritic cells, which are antigen-presenting cells. These dendritic cells are modified with lentiviral vectors to express a manmade minigene for select SARS-CoV-2 proteins (“Immunity and Safety of Covid-19 Sythetic Minigene Vaccine”). A lentivirus is an RNA virus that can transduce non-dividing and dividing cells, meaning that it can insert foreign genetic material into other cells (Li and Rossi).
Shenzhen Geno-Immune Medical Institute is also testing a vaccine called Pathogen-specific aAPC (“Safety and Immunity of Covid-19 aAPC Vaccine”). aAPC technology involves creating artificial antigen-presenting cells by attaching two to three signals to a vector that trigger T-cell responses (Neal). These signals include the major histocompatibility complex and the CD80 and CD86 proteins. The aAPCs in this vaccine are modified with a lentiviral vector to express a manmade minigene for select SARS-CoV-2 proteins (“Safety and Immunity of Covid-19 aAPC Vaccine”).
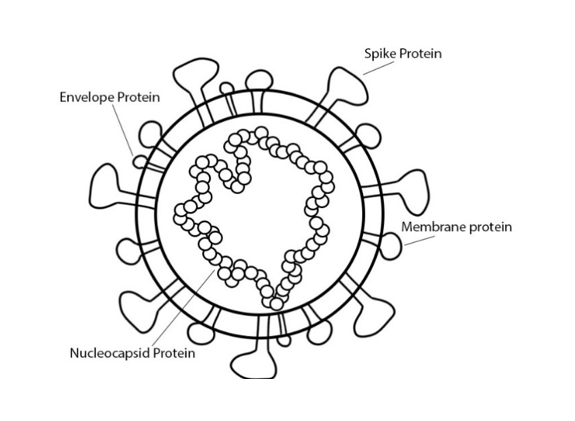
When will a vaccine be created?
The creation for a vaccine to combat the coronavirus is around 12-18 months away. While most vaccines take a decade or longer to be approved, scientists are working to develop a schedule for testing that will allow the process to be faster while still ensuring that the vaccine is safe and effective. The clinical trial that scientists are working to put vaccines through has four phases. Phase 1 will consist of a small group of healthy individuals and will focus on the vaccine’s safety and observing the immune responses that it causes at different doses. In Phase 2, hundreds of people will participate in a randomized, double blind, and placebo-controlled study where scientists will evaluate safety and efficiency, figure out the optimal dose, and generate the ideal vaccine schedule. The next part of the clinical trial is the regulatory review, where the organization in charge of approving new vaccines will review the information collected from the trial. The final phase includes post approval studies that monitor the effectiveness of the vaccine in real world conditions (Prichep).
“The creation for a vaccine to combat the coronavirus is around 12-18 months away.”
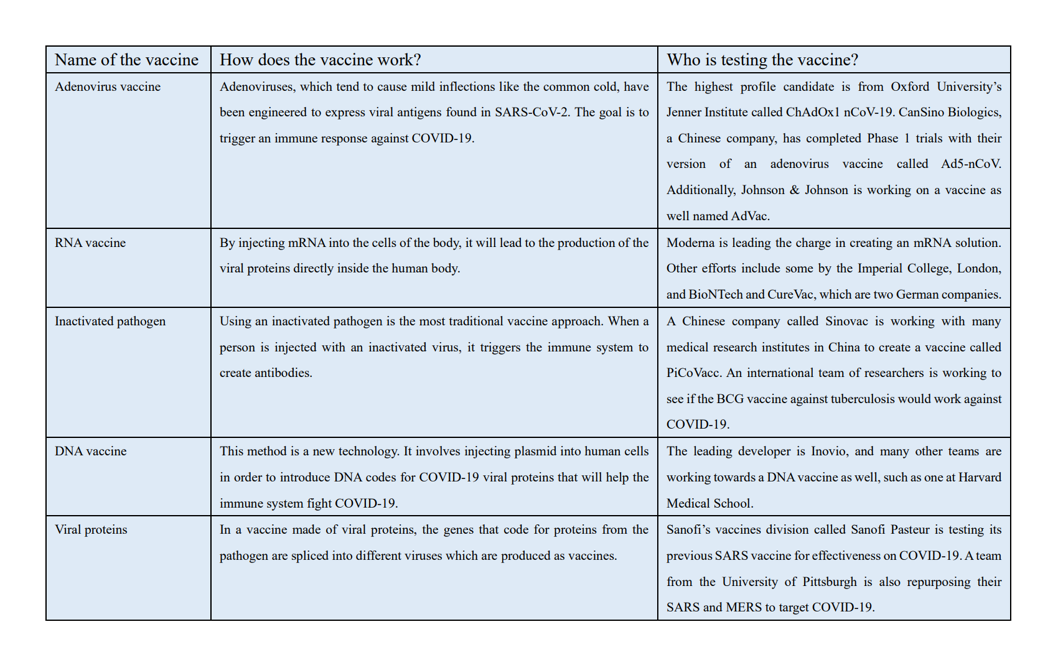
A major concern in the medical field is that, with different 100 treatments and vaccines working to be created at one time, we are wasting time and resources (Jasarevic). To solve this problem, the National Institutes of Health announced on April 17th a plan to organize clinical trials for the drugs and vaccines that are the highest priorities for testing and development. This initiative, called Acceleration COVID-19 Therapeutic Interventions and Vaccines (ACTIV), includes NIH, other U.S. government agencies, 16 pharma companies and biotechs, and the Foundation for the National Institutions of Health. The goal is the make the best use of the resources that NIH has by working with companies to evaluate data on early vaccine candidates and bring the ones with the most promise to NIH’s carefully designed clinical process. The best candidates will get priority for the $1.8 billion fund the Congress gave NIH for COVID-19 research, as well as access to NIH’s clinical trial network (Kaiser).
What will it take to get vaccines to the masses?
Even if a vaccine is created and approved in 12-18 months, researchers warn that it may not be possible to make the vaccine available to everyone. It is challenging to get vaccines to everyone who needs it because all countries will have to compete for the same medicine. Since pandemics tend to be the most severe in countries that house fragile and underfunded healthcare systems, there is a constant struggle between need and purchasing power when it comes to medicine. For example, in the 2009 H1N1 flu pandemic, vaccines were grabbed by the countries that could afford them and most of the developing countries who needed the vaccines just as much if not more were not able to purchase them. Experts also worry that major suppliers of vaccines such as India may use their production to help their 1.3 billion people before exporting it to other countries that do not have the capacity to produce vaccines at the same scale. Additionally, the resources needed for manufacturing the COVID-19 vaccine will have to be balanced against the need for other crucial vaccines, such as the influenza vaccine that is made in hundreds of millions of doses every year (Khamsi).
“…challenging to get vaccines to everyone who needs it because all countries will have to compete for the same medicine.”
About the Author
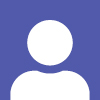
Calla O’Neil is a 16-year-old high school sophomore who attends Whittle School and Studios in Washington, DC. She is fascinated by global pandemics and international collaboration and was excited to combine these topics with her passion of writing in co-authoring this paper.
Ella Song is also a 16-year-old sophomore at Whittle School and Studios in Washington, DC. She has an interest in genetics and pathology that she enjoyed exploring for this paper.