Genomics: Insight
How Genomic Discoveries Are Shaping Global Food Security
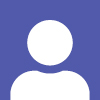
Research Question: How can discoveries in the field of genomics solve the global issue of food security?
Introduction
Genomics, the study of the genes in our DNA, has the potential to alleviate various global issues, including food insecurity. In 2024, there are up to 309 million people who face acute levels of food insecurity in countries with World Food Programme (WFP) operations<sup>1</sup>. The World Food Programme (WFP) is an organization within the United Nations (UN) that combats global hunger by providing life-saving food aid to those affected by conflict, disasters, and displacement while promoting long-term solutions like nutrition, agricultural support, and resilience to climate shocks<sup>7</sup>. Concerningly, by 2030, there are projected to be nearly 600 million people who are chronically undernourished<sup>2</sup>. As there is an ever-increasing number of people facing food insecurity, there is also an increasing need for genomic technology in agriculture to increase food supply. Some emerging solutions incorporate genomics and biotechnology to improve agricultural efficiency. Specifically, CRISPR-Cas 9 technology can be used to enhance crop yield and reduce the effects of both biotic and abiotic stresses, contributing to an overall improvement in global food security.
Genomics, the field of study of the genes in our DNA, has the potential to alleviate various global issues, among them food insecurity. Step by step, through safe and ethical practices of genomic technologies, we are inching closer and closer to a future where there is zero hunger.
Genomic Advances in Crop Improvement
The discovery and development of CRISPR-Cas 9—the genome editing technology that allows genetic information to be altered, deleted, or inserted at specific loci of a genome—has led to the
potential for its use in improving many different industries, including agriculture<sup>3</sup>. Single guide RNA (sgRNA) targets specific regions in the genome, which allows for precise mutations in crops. This specific targeting is crucial for manipulating desired traits such as increased yield or stress resistance. When sgRNA binds to its targeted DNA sequence, it guides the Cas 9 protein to create a double-strand break. During the repair process for this double-stranded break, there could be mutations through homologous recombination or non-homologous end joining. These mutations caused by sgRNA can lead to various differences in phenotypes, such as changes in plant height, crop yield, etc. depending on the targeted genes<sup>5</sup>. Essentially, the genetic editing of crops can create resistance to various environmental stressors that potentially decrease crop yield, enhancing crop yield and quality while decreasing reliance on pesticides, herbicides, and chemicals. A specific case study of a successful implementation is on a worldwide staple food: rice. Scientists used CRISPR-Cas 9 to edit a precise genome of rice, making it more resistant to biotic and abiotic stressors while facilitating the development of new varieties<sup>5</sup>.
Genome Editing for Resistance Against Abiotic and Biotic Stressors
Climate change is resulting in problems for rice farming. Severe weather conditions, for example, temperatures getting too hot or too dry, create difficult conditions for rice to grow. Biotic stresses, problems caused by living factors like pests and diseases, can be detrimental to rice plants. Abiotic stresses, problems caused by non-living factors, like drought and salinity affect how well rice plants grow. Two common diseases that harm rice plants are caused by the fungus Magnaporthe oryzae and the bacterium Xanthomonas oryzae. X. oryzae targets SWEET genes in rice; these genes help transport sugar in the plant, which the bacterium uses to grow<sup>5</sup>. To improve resistance to bacterial stress caused by X. oryzae, scientists targeted a specific
SWEET gene, OsSWEET13 by creating a sgRNA. They created two mutant lines of rice that used CRISPR to make deletions in the OsSWEET13 gene, using the Agrobacterium-mediated transformation method. The mutant rice plants showed significantly higher resistance to X. oryzae. In fact, the size of the damage caused by the disease was 90% less compared to regular rice crops<sup>4</sup>. An example of an improvement in rice’s tolerance of an abiotic stressor is salinity tolerance. Salinity tolerance refers to how well a plant can survive and thrive in salty soil or water. Oftentimes, conditions with high salinity can harm plants. The OsRAV2 gene is a specific gene in rice that helps it respond to salinity, essentially telling the rice plant how to respond when there is high or low salinity. Duan et al. focused on the promoter of the OsRAV2 gene. A promoter is a part of a gene that controls how much and when the gene is used, essentially acting as a switch that turns the gene on and off. There is a specific area in the promoter of OsRAV2 called the GT-1 region which helps the gene respond to salt. The researchers targeted the GT-1 region using sgRNA and created twelve different rice lines with changes in this GT-1 region. Out of these twelve varieties, two that did not contain the GT-1 region were selected as mutants and tested under high salinity conditions. The results were that the mutated rice varieties were not able to effectively produce the OsRAV2 gene in high salinity conditions, therefore showing that the GT-1 region is crucial for the proper functioning of the OsRAV2 gene under high salinity conditions<sup>8</sup>. Understanding the importance of the GT-1 region in rice salinity tolerance is crucial for the next step: developing rice varieties that have high salinity tolerance. This will improve food security, especially in regions where salinity is a problem. Similarly, promising results were found for other abiotic and biotic stresses.
Genomic Editing for Enhanced Crop Yield
CRISPR has also been used to improve the grain yield of rice. Genes that affect grain yield are quantitative trait loci (QTLs). They determine how well the plant grows and produces rice. Plant breeding before CRISPR involved breeders mixing different QTLs to find the optimal combination of improved rice plants. This involved cross-breeding different rice varieties with desirable traits in hopes of a better outcome. There were many downsides to this process, including lengthy breeding cycles, unpredictable outcomes, and inefficiency. The discovery of CRISPR allows for multiple QTLs to be targeted at once, precise modifications, and more predictable outcomes. In this case study, scientists targeted specific genes that influence yield in rice, including Gn1a, DEP1, GS3, and IPA1. Deletion and insertion mutations in Gn1a can lead to frameshifts which result in taller plants and larger panicles (flower clusters), resulting in approximately 90% more flowers than normal. Deletion mutations in DEP1 can result in shorter plants but increase the number of flowers by around 50%. Deletions in GS3 result in larger grains and longer awns (hairlike structures on the husk). Deletions and insertions in IPA1 can lead to frameshifts, which can potentially affect the number of tillers (side shoots) among other traits. The success rate of incorporating these mutations into the gene varies with the gene, with success rates of 42.5%, 67.5%, 57.5%, and 27.5% for Gn1a, DEP1, GS3, and IPA1, respectively<sup>5</sup>.
Societal and Environmental Implications
The results of this case study are promising, with CRISPR proving to be a useful force in transforming the agricultural industry through resistance against biotic and abiotic stressors, and increasing crop yield. However, with this rapid development, implications for society and the environment need to be considered. First, the rapid development of CRISPR could negatively impact the livelihoods of small-scale farmers who don’t have access to genomic technology and
are therefore unable to keep up with the times. This could lead to the potential widening economic disparity between small farms and large agricultural businesses. Given this information, it is crucial for policies and programs supporting technology adoption by small farmers to be put into place. Additionally, the use of CRISPR technology could have unintended consequences on the environment. The use of precision agriculture is associated with certain changes in local flora and fauna, and the cultivation of genetically modified (GM) crops may have harmful effects on the environment, soil, and water<sup>6</sup>. This emphasizes the importance of being cautious when cultivating GM crops, potentially choosing isolated areas where there is limited local biodiversity in order to protect the biodiversity of other areas. Nevertheless, ongoing research is needed to assess and address the implications of genomic interventions on the Earth’s biodiversity. Lastly, given the widespread societal skepticism and misinformation about GMOs, there is a need to address consumer perceptions and correct misconceptions. Through developing robust policies, we can ensure the safe use of genomic technologies while alleviating some consumer concerns about GMOs.
Conclusion
CRISPR technology has been successfully used to enhance and optimize certain traits of crops including resistance against biotic and abiotic stressors, improved yield, and nutritional enhancement. Genomic technology has a promising role in creating a future of resilient food systems. The future use of genomic technologies on crops could create high quantities of nutritionally dense foods, taking a step toward a world with no food insecurity. With the potential of genomic technologies to solve global food insecurity, I hope to see increased funding for genomic research in the agricultural industry. Step by step, through safe uses of genomic
technologies, we are inching closer and closer to a future of zero hunger. I chose this topic as the perfect combination of two issues I’m passionate about: food security and biotechnology.
References
- Ending hunger. (2024). World Food Programme. Retrieved October 29, 2024, from https://www.wfp.org/ending-hunger
- Food. (n.d.). United Nations. Retrieved October 29, 2024, from https://www.un.org/en/global-issues/food
- Eş, I., Gavahian, M., Marti-Quijal, F. J., Lorenzo, J. M., Khaneghah, A. M., Tsatsanis, C., ... & Barba, F. J. (2019). The application of the CRISPR-Cas9 genome editing machinery in food and agricultural science: Current status, future perspectives, and associated challenges. Biotechnology Advances, 37(3), 410-421.
- Zhou J H, Peng Z, Long J Y, Sosso D, Liu B, Eom J S, Huang S, Liu S Z, Vera Cruz C, Frommer W B, White F F, Yang B. (2015). Gene targeting by the TAL effector PthXo2 reveals cryptic resistance gene for bacterial blight of rice. Plant J, 82(4): 632–643.
- Romero, F. M., & Gatica-Arias, A. (2019). CRISPR/Cas9: development and application in rice breeding. Rice Science, 26(5), 265-281.
- Tsatsakis, A. M., Nawaz, M. A., Tutelyan, V. A., Golokhvast, K. S., Kalantzi, O. I., Chung, D. H., ... & Chung, G. (2017). Impact on environment, ecosystem, diversity and health from culturing and using GMOs as feed and food. Food and Chemical Toxicology, 107, 108-121.
- Who we are. (n.d.). World Food Programme. Retrieved November 22, 2024, from https://www.wfp.org/who-we-are
- Duan Y B, Li J, Qin R Y, Xu R F, Li H, Yang Y C, Ma H, Li L, Wei P C, Yang J B. (2016). Identification of a regulatory element responsible for salt induction of rice OsRAV2 through ex situ and in situ promoter analysis. Plant Mol Biol, 90(1): 49–62.
About the Author
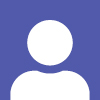
Jasmine Zhao is a high school junior at Hong Kong International School in Hong Kong. She is particularly interested in biotechnology and its applications in solving global issues.
Mentor: Mrs. Dee Mulligan Affiliation: Hong Kong International School.